Core-collapse supernovae
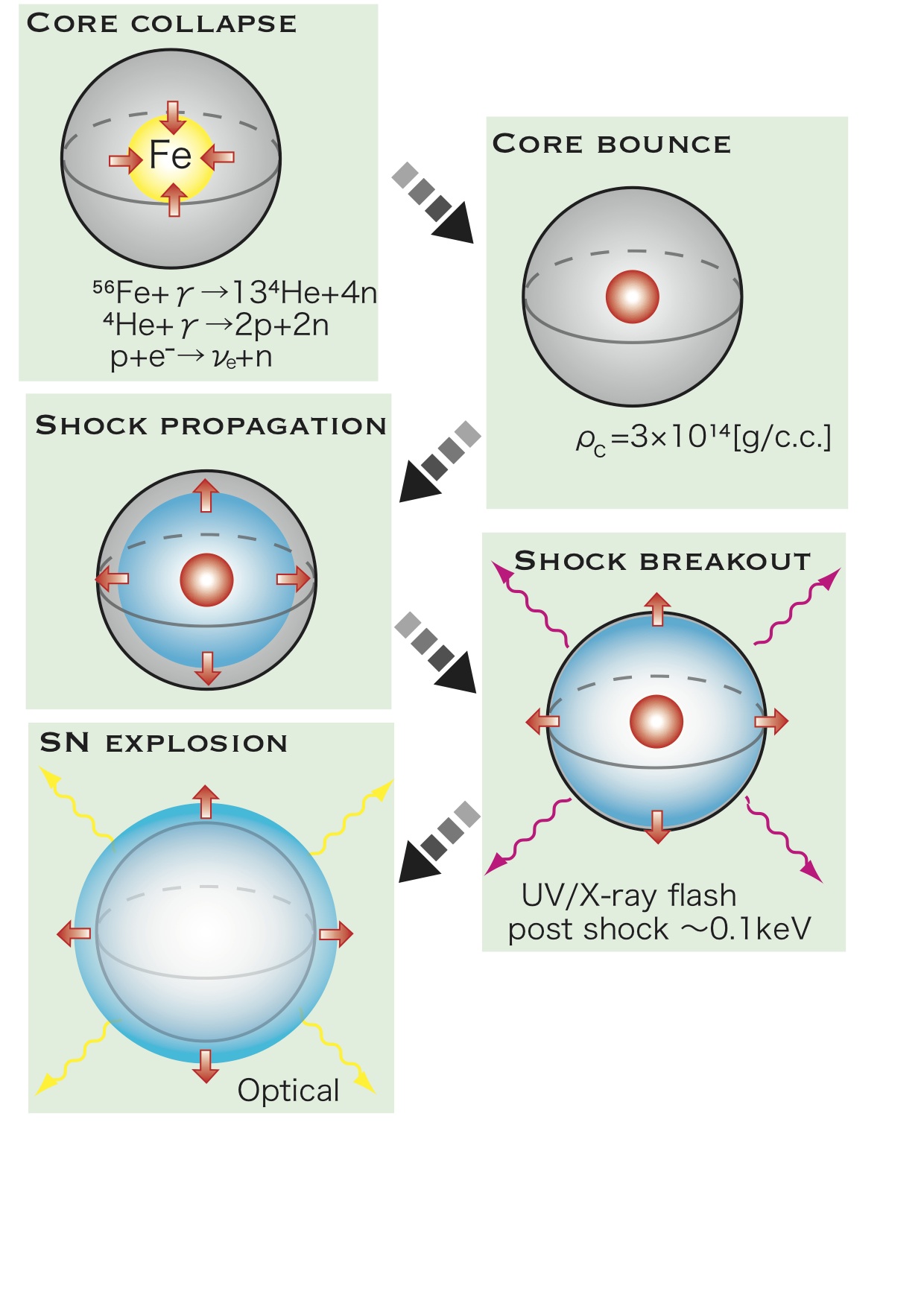
Central engine model for extra-ordinary supernovae
One of the successes of the above modern transient survey programs is the discovery of a special class of SNe characterized by their extreme brightness. They are now called superluminous supernovae. Their extreme brightness makes it possible for us to find superluminous supernovae even in distant galaxies. However, the problem is that the energy source responsible for their brith thermal emission has yet to be specified. One of the plausible scenarios is the central engine scenario, in which the supernova produce a central compact object (highly-spinning magnetized neutron star, black hole accretion disk, or whatever) and it deposits an additional energy into the supernova ejecta. Because of the energy injection from the central energy source, the supernova ejecta are energized and give rise to bright thermal emission. I'm currently working on the numerical computation by using parallel supercomputers to reveal what would happen in this system and find observational signature distinguishing the central engine-powered SNe from other scenarios.
Interacting supernovae
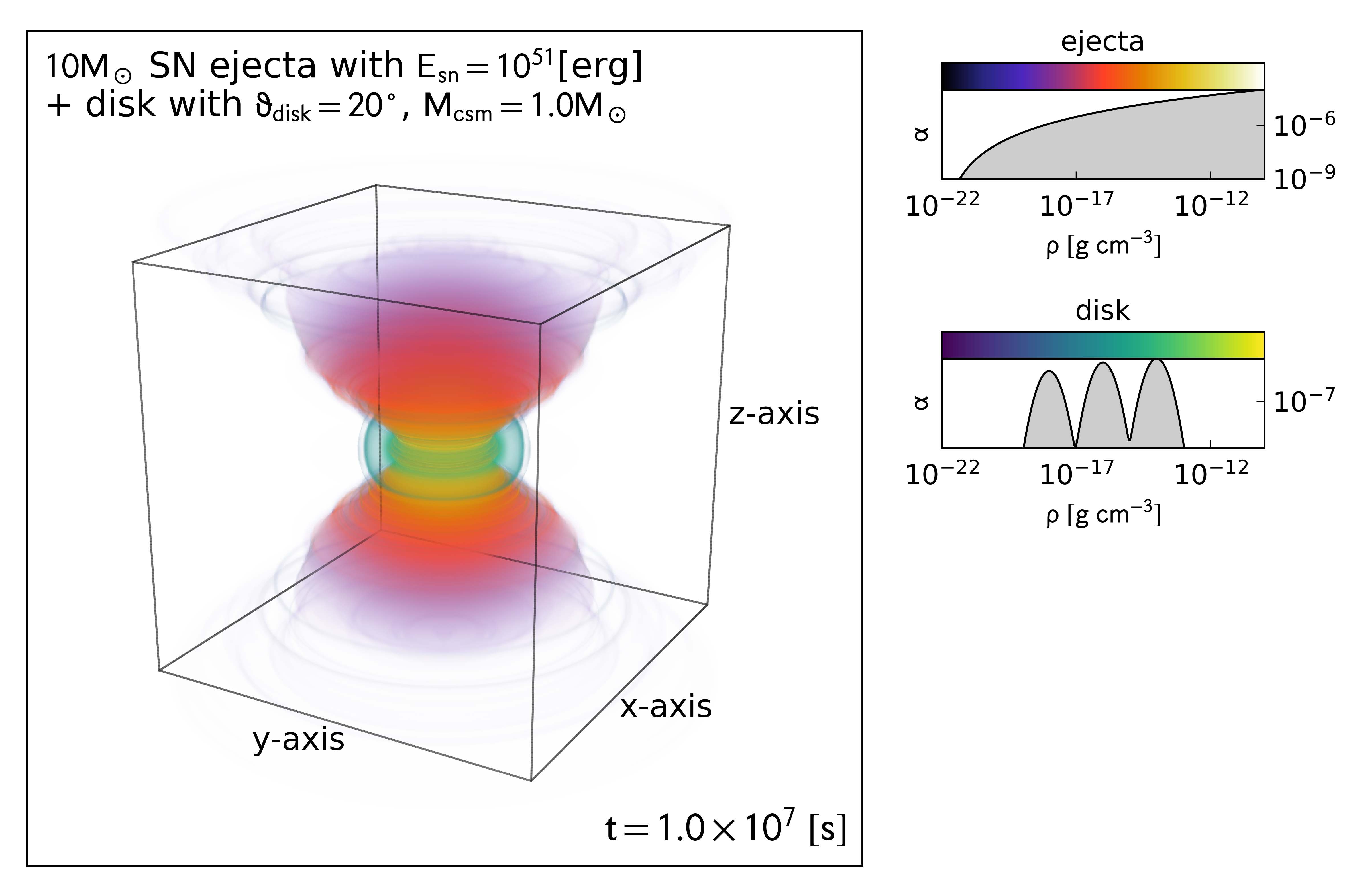
A specific class of CCSNe show an evidence of a dense gas surrounding the progenitor star, i.e., the so-called circum-stellar medium (CSM). The presence of CSMs could be infered by a characteristic spectral feature, narrow absorption/emission lines superposed on broad P-cygni lines, which is usually observed in sueprnova spectra. Narrow spectral lines indicate a slowly moving (~10-100km/s) gas is ahead of fast SN ejecta traveling at ~10,000km/s. SNe with interaction features are called interacting SNe or interaction-powered SNe and comprise a sub-class of SNe. In terms of spectroscopic classification of SNe, they are classified into type IIn and Ibn SNe, depending on the presence/absence of hydrogen and helium spectral features. Even for type Ia SNe, which are thermonuclear explosions of white dwarfs, there are some events exhibiting interaction feature and they are called type Ia-CSM.
One of the impotant aspects of the ejecta-CSM interaction is that the collision between the two flow with different velocities can dissipated the kinetic energy of the flows as thereby serves as a power source for bright thermal emission. In fact, some superluminous SNe, such as SN 2006gy, show spectral features indincating the presense of a massive CSM. Such massive CSMs in the vicinity of exploding stars should have been produced immediately before the stellar explosion. However, the problem is that we currently do no know how and when exactly such massive CSMs are produced. There are some proposed mechanisms (binary interaction, commomn envelope, wave-driven eruptive mass-loss, and so on) for such extensive mass-loss processes prior to stellar explosions.
Supernova shock breakout
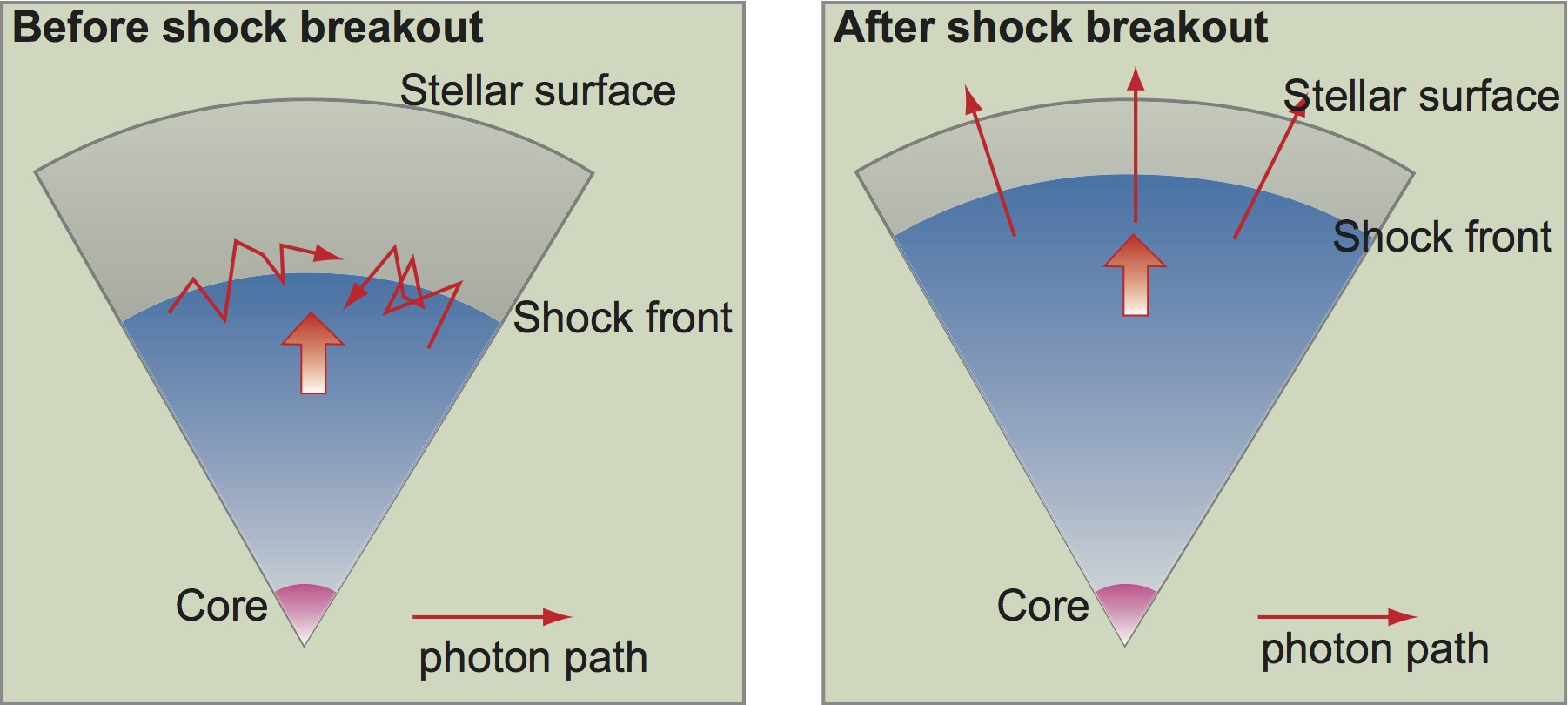
Gamma-ray bursts
Gamma-ray bursts (GRBs) are enigmatic bursts of gamma-rays found on the celestial sphere. Space gamma-ray and X-ray observatories routinely find these spontaneous bursts at a rate of 1 event per day. Currently, they are thought to be violent explosions happening in distant galaxies. They are classified into two broad categories, long- and short-GRBs, according to the duration of the prompt gamma-ray emission. The most plausible scenarios for long- and short-duration events are massive star's death and the coalescense of a neutron star-neutron star binary. Highly energetic photons can be produced by ultra-relativistic jets driven by these violent events. However, there are still remaining promlems, such as, what is the mechanism driving the jet and how gamma-rays are created.low-luminosity GRBs
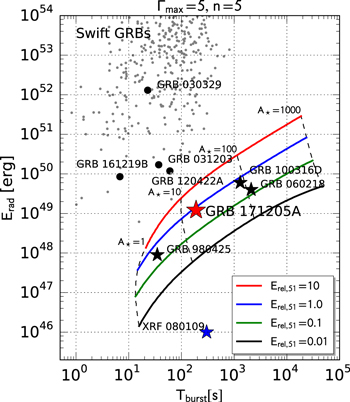
Others
chemical evolution of the Galaxy
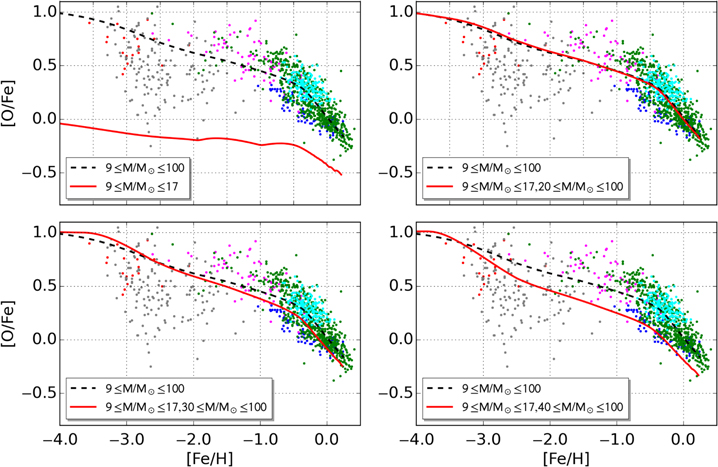